AKA Citric Acid Cycle AKA Krebs Cycle, TCA = Tricarboxylic Acid
Acetyl-CoA can enter the TCA cycle, which occurs inside the mitochondrial matrix in eukaryotes and in the cytosol of prokaryotes. The goal of this cycle is to generate high enery electron carriers, two NADH’s and one FADH2, as well as energy in the form of one GTP, from the oxidation of acetyl-CoA. It’s called a cycle because a number of substrates and products of the cycle are reused over and over, specifically the main 4-carbon skeleton. The overall digram of TCA is shown below.
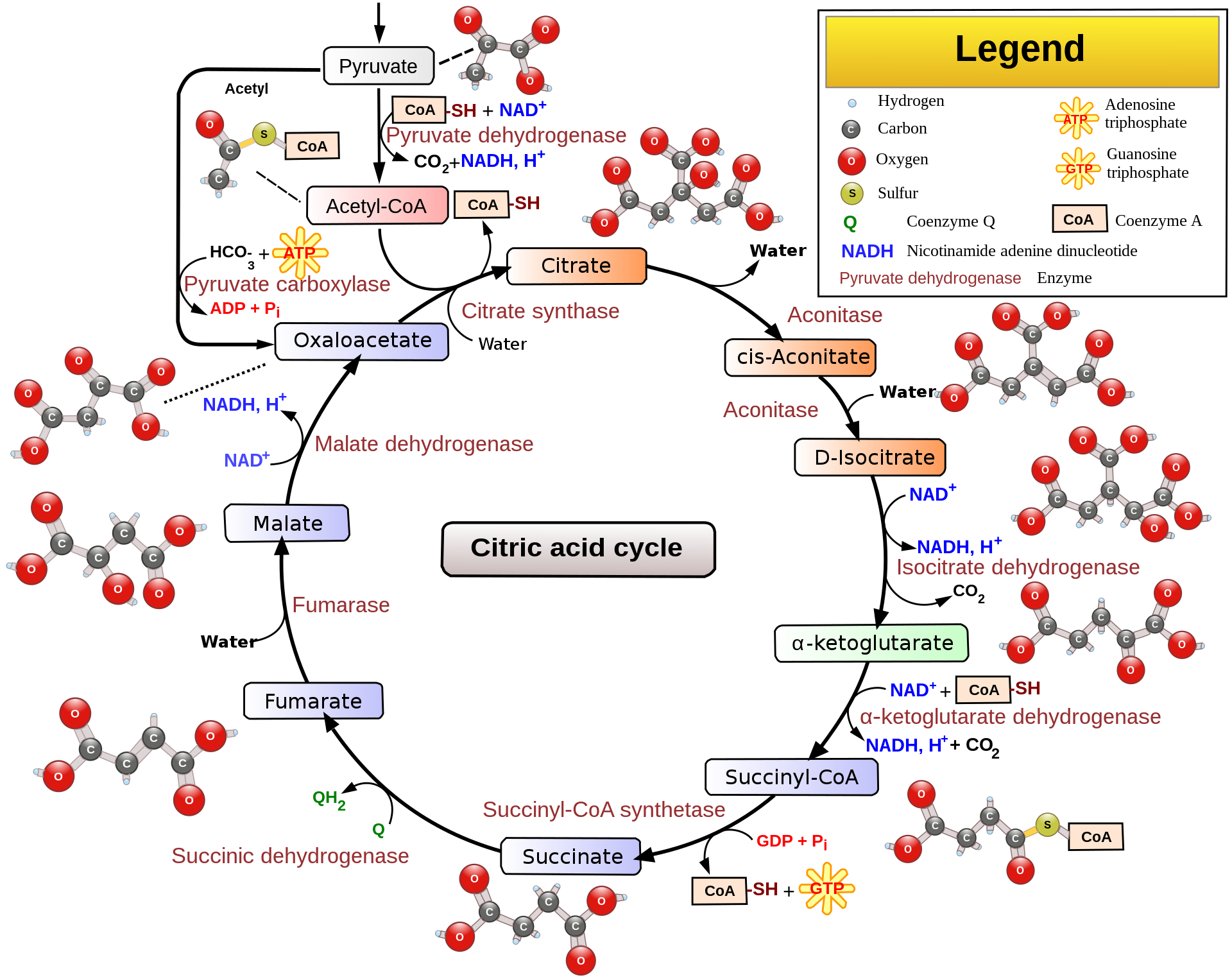
You may notice from the diagram above that oxygen isn’t present. So why is TCA considered an aerobic-only process? Under anaerobic conditions, the cycle will not run due to a buildup of NADH and FADH2, which inhibit the cycle. The electron transport chain does not run in the absence of oxygen, which is why NADH and FADH2 build up.
Since TCA is a cycle, the last step (10th step) is also the 0th step. This is the entry of acetyl-CoA into the cycle, where it couples with a molecule of oxaloacetate to form citrate. Citrate is a tricarboxylic acid and is by convention defined as the starting point of the cycle. In this step, acetyl-CoA and oxaloacetate undergo first a condensation reaction (reaction in which two or more molecules combine, in this case specifically an aldol condensation) to form citryl-CoA, an intermediate. Hydrolysis (cleavage of bonds by water) of citryl-CoA, which is energetically favorable, then yields citrate and CoA-SH, which is released into the matrix. This overall reaction is catalyzed by citrate synthase(synthases are enzymes that form new covalent bonds without needing significant energy input). In the 1st step, citrate is turned into cis-Aconitate by the enzyme Aconitase through a dehydration reaction. Then the 2nd step turns cis-Aconitate into isocitrate through a hydration reaction with one free water molecule, again with the enzyme Aconitase. The overall effect of these two steps is an isomerization, shown below.

The isomerization above was necessary to facilitate the oxidative decarboxylation found in the 3rd step, in which isocitrate is oxidized to oxalosuccinate by the enzyme isocitrate dehydrogenase, producing an NADH in the process (NADH is worth 2.5 ATP’s in oxidative phosphorylation). Then in the 4th step, oxalsuccinate is decarboxylated to produce α-Ketoglutarate, releasing CO2 in the process. The enzyme is again isocitrate dehydrogenase. Importantly, isocitrate dehydrogenase is the rate-limiting enzyme of the entire TCA cycle.

In the 5th step, α-Ketoglutarate undergoes oxidative decarboxylation by the α-Ketoglutarate dehydrogenase complex, which is similar to the pyruvate dehydrogenase complex, to form succinyl-CoA. CoA and NAD+ are present in the matrix. The second NADH is generated and the second CO2 is released. Past this step, the cycle is irreversible. Quick aside: dehydrogenases are a subtype of oxidoreductases (enzymes that catalyze an oxidation-reduction reaction). Dehydrogenases transfer a hybride ion (H-) to an elctron acceptor, usually FAD or NAD+.

In the 6th step, succinyl-CoA undergoes a substrate level phorphorylation to turn one GDP molecule to a GTP molecule with a free-floating phosphate. The thioester bond on succinyl-CoA is hydrolyzed to form succinate and CoA-SH, which is coupled to the phosphorylation. The enzyme responsible is succinyl-CoA synthetase. The hydrolysis of thioester bonds is accompanied by a significant release of energy. GTP and ADP can be freely converted to GDP and ATP by the enzyme nucleosidephiphosphate kinase. This is the only direct ATP production by the TCA cycle.

Succinate is oxidized by the enzyme succinate dehydrogenase to form fumarate in the 7th step. This is step also forms FADH2, which is from the reduction FAD. Succinate dehydrogenase is considered a flavoprotein becuase it is covalently bonded to FAD, which is the electron receptor. This step is also the only one that doens’t occur in the mitochondrial matrix, but instead occurs on the inner membrane. This way, the two electrons are transferred to QH2 during Complex II of the electron transport chain, which redeems them for 1.5 ATP. The reducing power of succinate is not great enought to reduce NAD+.

Malate is formed in the 8th step when fumarase catalyzes the hydrolysis of the alkene bond in fumarate. H20 is necessary for this reaction. In the 9th step, oxaloactetate is reformed by the oxidation of matle by the enzyme malate dehydrogenase. One NADH+H+ is also reduced from NAD+ in this step. This is the third NADH formed in the cycle.

You can use this mnemonic to remember the substrates of the TCA Cycle: Please, Can I Keep Selling Seashells For Money, Officer? Pyruvate, Citrate, Isocitrate, α-Ketoglutarate, Succinyl-CoA, Succinate, Fumarate, Malate, and Oxaloacetate.
So how is the TCA cycle regulated? There are three key enzymes that are regulated: citrate synthase, isocitrate dehydrogenase, and α-Ketoglutarate dehydrogenase complex. Citrate synthase is allosterically inhibited by ATP and NADH, as well as by citrate and succinyl-CoA. Remember, allosteric inhibition is regulation by binding at a site on the enzyme that isn’t the active site. Isocitrate dehydrogenase is inhibited by ATP and NADH but is also allosterically activated by ADP and NAD+, which increase its affinity for substrates. α-Ketoglutarate dehydrogenase complex is inhibited by succinyl-CoA and NADH, as well as ATP. The complex is activated by ADP and calcium ions. Overall, this makes sense because high levels of ATP and NADH indicate an energy surplus, in which case TCA doesn’t need to run as much. When energy is rapidly consumed, such as during exercise, ADP and NAD+ levels are high, and TCA would need to run rapidly to meet energy demands.